Elastography methods can measure how stiff or rigid certain organs or tissues inside the body may be. More specifically, elastography methods measure the shear modulus of these tissues, which is a measure of resistance to shape change, or stiffness. Abnormal stiffness can be indicative of diseases such as liver fibrosis, breast and prostate cancer, Alzheimer’s disease, etc.
While several different methods based on MRI, ultrasound imaging and even mechanical testing can evaluate the shear modulus, they tend to all give different numbers that do not agree well with each other. It is increasingly recognized that the shear modulus value that is obtained may depend on how fast (or slow) one shakes the tissues in the measurement process. Different methods tend to operate at different frequencies, for example, MR Elastography (MRE) is usually performed with a shaking device that operates at about 50 or 60 Hz, while ultrasound Shear Wave Elastography (SWE) typically operates over a range from about 100 to 2000 Hz, roughly. We studied the frequency dependences of the shear modulus because: 1) they can help explain why different methods give different numbers, but also 2) because we hope that measuring how stiffness varies with frequency might prove to be a way of characterizing and diagnosing diseases.

Mechanical devices called rheometers, Fig. 1, can measure stiffness but they typically do so only on tiny samples, such as disks only a few mm in thickness. We modified our rheometer so it could handle larger samples (Fig. 1), large enough that ultrasound SWE could be performed on them. Figure 2 shows the shear modulus values (called G) measured with the rheometer at low frequencies (0 to 100 Hz, in blue) and with SWE at higher frequencies (100 to 1000 Hz, in red). The sample was a piece of gelatin, which is a Jello-like material (yet stiffer than Jello, maybe more like a piece of Jello forgotten in the refrigerator for a while). A clear message from Fig. 2 is that the value of the shear modulus, G, very much depends on the frequency employed to measure it. Worth noting, the shear modulus in Fig. 2 is about 50% higher at 500 Hz (typical for ultrasound elastography) than at 60 Hz (typical for MR elastography), which helps explain discrepancies between imaging modalities.
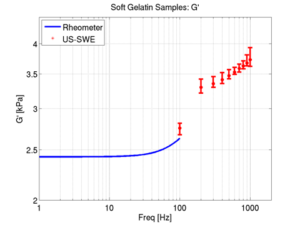
Ultrasound measurements similar to those from Fig. 2 were also performed on ex vivo liver bovine tissues, as shown in Fig. 3. The real part of G, which is what elastography methods typically try to measure, is shown in Fig. 3a. In contrast, the imaginary part of G as shown in Fig. 3b, tends to be much more difficult to measure reliably, so much so that regular elastography methods do not typically attempt to measure it. Estimates at three different locations in the liver tissues are shown in Fig. 3 using different line colors.
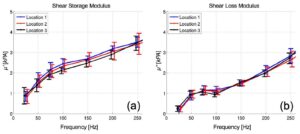
Note that bovine liver exhibits significant dispersion effects over the 25-250 Hz range displayed in Fig. 3: a 4-fold increase in shear storage modulus and a 10-fold increase in shear loss modulus. In summary, the value of the shear modulus being measured depends on the frequency of the mechanical oscillation employed to measure it, due to dispersion effects. While these effects have long been neglected in the elastography literature, they should be taken into account whenever data from modalities that operate at different frequencies should be reconciled.
- Yengul SS, Barbone PE, Madore B. Dispersion in tissue-mimicking gels measured with shear wave elastography and torsional vibration rheometry. Ultrasound in Medicine and Biology 2019; 45: 586-604. PMCID: PMC6325023
- Yengul SS, Barbone PE, Madore B. Application of a forward model of axisymmetric shear wave propagation in viscoelastic media to shear wave elastography. J Acoust Soc Am 2018; 143: 3266-3277. PMCID: PMC5986577
- Yengul SS, Barbone PE, Madore B. Wide-band dispersion measurements in ex-vivo bovine liver using ARFI-SWE based Shear Wave Rheometry with metrics for estimating tissue anisotropy and inhomogeneity.
Yengul SS, Barbone PE, Madore B. Wide-band dispersion measurements in ex-vivo bovine liver using ARFI-SWE based Shear Wave Rheometry with metrics for estimating tissue anisotropy and inhomogeneity.
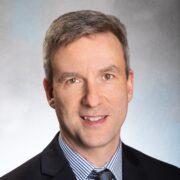
Associate Professor of Radiology
Bruno obtained a B.Sc. in Physics from Laval University in Québec City, and a Ph.D. in medical biophysics from the University of Toronto. He also performed postdoctoral studies at Stanford University, in fast Magnetic Resonance Imaging (MRI).
His main research expertise lies in the development of novel acquisition and image reconstruction strategies for Magnetic Resonance Imaging. He also has strong interest in ultrasound imaging, and in combining it with MRI. More generally, much of the work in the ALMA lab involves encoding useful information that relates to relaxation, dynamic motion, diffusion and/or thermometry into MR or ultrasound signals in novel ways. This information is then recovered at the image reconstruction stage, to generate images that are better and/or richer in terms of information content than what would otherwise have been the case.
Bruno is Deputy Editor and ‘Senior Deputy Editor for Physics and Techniques’ for the Journal of Magnetic Resonance Imaging (JMRI), one of two official journals of the ISMRM. He was awarded a ‘Distinguished Investigator Award’ by the Academy of Radiology Research in 2016, and a ‘Young Investigators’ Moore Award’ by the ISMRM in 1999.